Permian Magmatism
The latest Carboniferous marked the onset of bimodal magmatism in SW England characterised by ultramafic lamprophyre dykes and, slightly later, a Permian felsic granite batholith with associated quartz-porphyry dykes. The earliest lamprophyric rocks are intruded during the Stephanian into D3 extensional structures (Alexander and Shail, 1996). Later, Permian granite magmatism emplaced the Cornubian Batholith, exposed at surface as a suite of plutons, into the Devonian-Carboniferous sedimentary succession. The Cornubian Batholith comprises granites and quartz-feldspar porphyry dykes (locally referred to as “elvans”) which are illustrated in Figure 1.
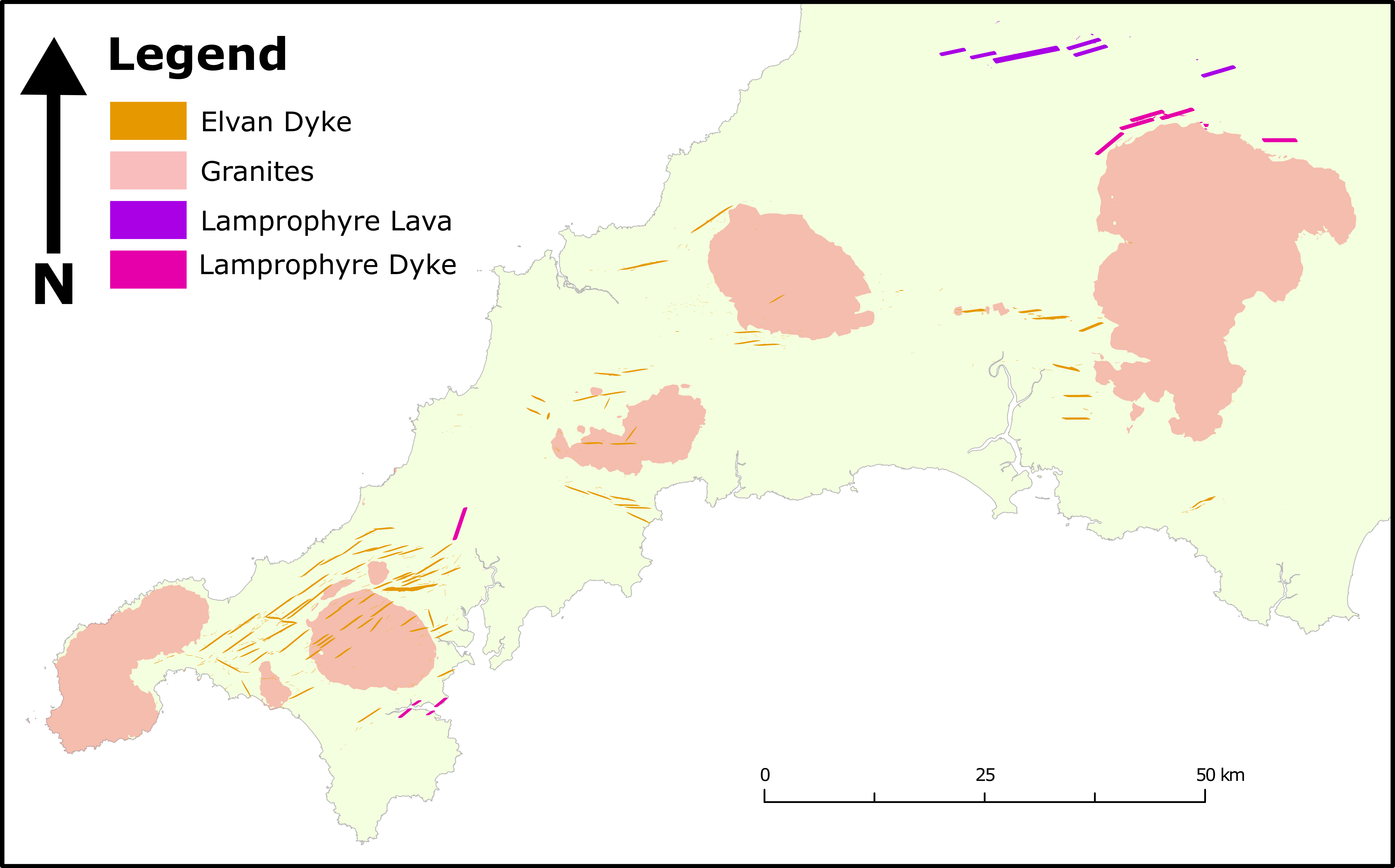
The surface crop of the granite plutons have been studied extensively. Poor outcrop exposure and various mapping techniques have led to contrasting interpretations of these plutons. In this section, the most up-to-date mapping and interpretation by Simons et al. (2016, 2017) is presented as the state-of-the-art. The work by Simons et al. (2016) presents a revised geological classification of the granite plutons based on previous mapping which has been augmented through new geochemical, mineral and field evidence. Further work by Simons et al. (2017) helps elucidate magma fractionation and the relationships between different granite classes identified in Simons et al. (2016).
Lamprophyres
Ultramafic lamprophyre intrusions ranging from olivine-basalt, minette (K-feldspar > plagioclase) to biotitic-microsyenite compositions occur across the SW peninsula (Edmonds et al., 1968; Edwards, 1999; Leveridge et al., 1990, 2002; McKeown et al., 1973). The “Exeter Volcanic Rocks” is a term used to describe significant occurrences of lamprophyric and basaltic dykes, sills and lava flows associated with extensional structures west of Exeter (Edwards, 1999). Lamprophyric dykes are notable further west such as the Pendennis, Mawnan, Treliske, Mountjoy and Furzehill Bridge lamprophyres, however, extrusive rocks are not found in these areas (Leveridge et al., 1990, 2002; Reid and Scrivenor, 1906), most likely because of erosion. The mafic melt is considered to have a mantle source with only minor crustal contamination or mixing with contemporaneous felsic melt (Leat et al., 1987; Simons et al., 2017). It is however, postulated that minette compositions may be achieved from lamproite magmas mixing with >30% felsic crustal melt (Prelević et al., 2004). Geochronological data for lamprophyric intrusive and extrusive bodies are summarised in Table 1.
Rock Type | Locality | Analytical Technique | Age (Ma) | Refs. | |
---|---|---|---|---|---|
Exeter Volcanic Rocks | Basalt | Dunchideock [SX 876 873] | K-Ar whole rock | 287 ±11 | 2 |
Minette | Killerton [SS 975 005] | K-Ar whole rock | 285 ±6 | 1 | |
Minette | Killerton [SS 975 005] | K-Ar biotite | 283 ±7 | 4 | |
Minette | Killerton [SS 975 005] | Ar-Ar biotite | 290.8 ±0.8 | 6 | |
Microsyenite | Knowle Hill | Ar-Ar biotite | 281.8 ±0.8 | 6 | |
Intrusive Lamprophyres | Minette | Bridford [SX 8263 8752] | K-Ar biotite | 300 ±4.6 | 3 |
Minette | Chyweeda [SW 613 326] | K-Ar phlogopite | 292.9 ±3.4 | 3 | |
Kersantite | Fremington | Ar-Ar plagioclase | 292.4 ±7.1 | 5 | |
Helford [SW 753 265] | Ar-Ar phlogopite | 287.59 ±0.68 | 7 | ||
Fisherman’s / Frenchman’s Creek [SW 749 258] | Ar-Ar phlogopite | 284.38 ±1.07 | 7 | ||
Pendennis Point [SW 766 601] | Ar-Ar phlogopite | 292.15 ±1.5 | 7 | ||
Trelissick [SW 827 316] | Ar-Ar phlogopite | 286.9 ±1.54 | 7 | ||
Holywell Bay [SW 834 387] | Ar-Ar whole-rock | 262.1 ±1.3 | 7 |
The geochronology data are latest Carboniferous to Early Permian age and indicates that mafic magmatism was coeval with granite magmatism and intrusion of the main batholith (see Table 2; Chesley et al., 1993; Chen et al., 1993; Dupuis et al., 2015). Spatially, lamprophyres do not occur over or within the batholith and a “shadow zone” exists suggesting lower crustal melting may have precluded the assent of mafic magma and resulted in hybridisation of the two melt components as part of the batholith (Shail et al., 2014). However, this does not preclude known lamprophyres to be altered by later mineralisation events (e.g. Holywell Bay; Dupuis et al., 2015).
Granite Magmatism
Granite plutons characterise many of the topographic highs across Cornwall and Devon such as Dartmoor and Bodmin Moor. These plutons were intruded into the Devonian-Carboniferous sedimentary succession during erosion, extension and exhumation of the Variscan orogen (Shail and Leveridge, 2009). The region has 6 major plutons, Land’s End, Tregonning-Godolphin, Carnmenellis, St Austell, Bodmin and Dartmoor with numerous smaller exposures, and occur in an approximately ENE-WSW trend, offset by NW-SE dextral strike-slip faults (Leveridge et al., 2002; Scrivener, 2006). These plutons are generally poorly exposed, with most outcrops forming weathered hilltops (tors) or are exposed in quarries. Limited exposure exists from underground mine workings whilst the Land’s End Granite, and a short section of the Tregonning-Godolphin Granite, are the only plutons with coastal outcrops which provide high-quality exposure. Much of the unexposed inland areas are mapped from field brash or “float” which is not in-situ. Simons et al. (2016) classified the plutons into 5 granite classes (G1-G5) and mapped the spatial distribution of these, and various subclasses over the region; illustrated in Figure 2.
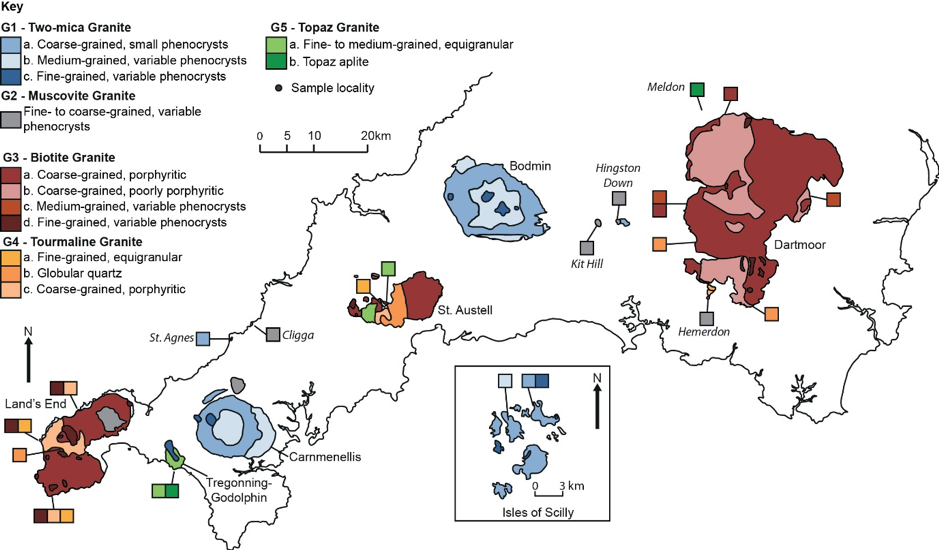
The interpretations by Simons et al. (2016) are based on whole-rock geochemical analyses and mineralogical data supported by geochronology and field-evidence where permissible. It should be noted that the whole-rock geochemistry and mineralogy is only collected from areas of outcrop and thus is limited to exposed areas. Therefore, whilst a robust classification can be made for known outcrops, much of the spatial distribution is inferred and harmonised with previous mapping.
The classification reconciled many other previous works that often focussed on a single pluton such as that of Booth and Exley (1987), Knox and Jackson (1990), Manning et al. (1996), Exley (1996) and Powell (2004). The major revisions made by Simons et al. (2016) were the classification of two-mica (G1) and muscovite (G2) granites and the grouping of Li-mica and tourmaline granite types into a single tourmaline (G4) class. The G4 class is contrary to the work by Manning et al. (1996) who considered Li-mica and tourmaline granites as geochemically distinct. The work also reflected the separation of two major magmatic stages that intruded G1 and G2 before G3 to G5; geochronology to support this is presented in Table 2. The timing of these magmatic events is supported and developed further by Tapster (2019, in preparation) and linked to the timing of lamprophyre intrusion.
Granite pluton/stock | Lithofacies | Locality | Method | Age ± 2σ Ma | Source |
---|---|---|---|---|---|
Land’s End | CGMBG | Lamorna Cove Quarry | U-Pb monazite | 274.7 ±0.4 | 4 |
CGMBG | Newmill Quarry | U-Pb monazite | 274.9 ±0.5 | 2 | |
CGMBG | Cripplesease | U-Pb monazite | 277.1 ±0.4 | 4 | |
FGBG | Castle-an-Dinas Quarry | U-Pb monazite | 276.7 ±0.4 | 4 | |
FGBG | Castle-an-Dinas Quarry | U-Pb xenotime | 279.3 ±0.4 | 2 | |
FGBG | Castle-an-Dinas Quarry | U-Pb zircon | 300 ±5 | 5 | |
FGBG | Polgigga | U-Pb monazite | 274.4 ±0.4 | 4 | |
Carnmenellis | CGMBG-SM | Carnsew Quarry | U-Pb monazite | 293.1 ±1.3 | 3 |
CGMBG-SM | Carnsew Quarry | U-Pb monazite | 293.7 ±0.6 | 2 | |
CGMBG-SM | Carn Brea | U-Pb monazite | 292.0 ±1.0 | 4 | |
FGBG | Boswyn | U-Pb monazite | 281.7 ±0.8 | 4 | |
CGMBG | Rosemanowes Quarry | U-Pb zircon | 313 ±3 | 5 | |
St Austell Granite | CGMBG | Luxulyan Quarry | U-Pb monazite | 280.6 ±0.7 | 3 |
U-Pb monazite | 281.8 ±0.4 | 2 | |||
U-Pb zircon | 305 ±5 | 5 | |||
Bodmin Moor | CGMBG-SM | U-Pb monazite | 291.4 ±0.8 | 2 | |
CGMBG | De Lank Quarry | U-Pb zircon | 305 ±4 | 5 | |
Dartmoor | CGMBG | Pewtor Quarry | U-Pb monazite | 281.0 ±0.6 | 4 |
CGPMBG | Haytor Quarry | U-Pb monazite | 285.3 ±0.6 | 4 | |
CGMBG | Yellowmeade Farm | U-Pb monazite | 278.2 ±0.8 | 2 | |
FGBG | Throleigh Common | U-Pb monazite | 286.2 ±1.0 | 4 | |
St Michael’s Mount | CGMBG-SM | Ar-Ar | 281.4 ±1.5 | 3 | |
Tregonning-Godolphin | FGBG | Godolphin Granite | Ar-Ar muscovite | 281.6 ±1.6 | 4 |
Topaz | Tregonning Granite | Rb-Sr whole rock | 280 ±4 | 1 | |
Topaz | Tregonning Granite | Ar-Ar zinnwaldite | 281.0 ±1.3 | 4 | |
Carn Marth | CGMBG-SM | Carn Marth Quarry | U-Pb monazite | 288.0 ±1.0 | 4 |
St Agnes | CGPMBG | Cameron Quarry | Ar-Ar muscovite | ≥278 | 3 |
Cligga Head | CGPMBG | Cligga Head | Ar-Ar muscovite | 279.9 ±0.8 | 3 |
Castle-an-Dinas | FGBG | Castle-an-Dinas | Rb-Sr whole rock | 270 ±2 | 1 |
FGBG | Castle-an-Dinas | Ar-Ar muscovite | ≥273 | 3 | |
Kit Hill | CGMBG-SM | Ar-Ar muscovite | 284.3 ±1.0 | 3 | |
Hingston Down | FGBG | Ar-Ar muscovite | 283.1 ±1.0 | 3 | |
Gunnislake | CGPMBG | Old Gunnislake Mine | Ar-Ar muscovite | 283.1 ±0.9 | 2 |
The work by Simons et al. (2016) provides an excellent foundation for comparing granitic rocks from across the batholith. It provides an insight into the internal variations of plutons, but also demonstrates the intra-class variability, primarily based on mineralogical analyses, within each defined class. The classification provides a contemporary, regionally consistent and up-to-date framework for granite classification.
Granite at Depth
The interpretations by (Simons et al., 2016) of the outcrop of the granite are considered robust, however, there is a significant assumption that the surface exposure of the granite batholith is representative of what lies at depth. Whilst the classification of different granite types within the batholith remains a difficult problem to resolve, some estimates can be placed on the shape and volume of the granite mass through geophysical interpretation.
Bott et al. (1958) demonstrated through gravity modelling that the granite plutons were linked in the subsurface and more extensive modelling showed that the batholith is approximately tabular (Willis-Richards and Jackson, 1989). Gravity modelling has been applied in various guises to define the extent of the granite at depth (Beer et al., 1975; Rollin, 1988; Rollin et al., 1982; Tombs, 1980, 1977), however, the modelling of the granite surface by Willis-Richards and Jackson (1989) provided a regional model for the upper surface of the granite, shown in Figure 3. There have been few occasions where boreholes have been drilled to validate these various models of the granite surface at depth, however, previous holes drilled based on smaller predictions include the intersection of a granite body at Bosworgey [SW 58060 33670] approximately 173 m (prediction; 350 m) and the failed intersection at Parbola [SW 61570 36370] where the hole stopped at 665.5 m following a predicted intersection at 540 m (Burley et al., 1978). When compared to depths in the regional surface model generated by Willis-Richards and Jackson (1989), these values indicate deeper values of 545 m and 530 m for Bosworgey and Parbola, respectively, and that they lie proximal to a sharp edge to the batholith. Therefore, it can be considered that current gravity modelling, either regional or locally, is insufficient. To-date, no new gravity measurements have been acquired and significant scope remains for a high-resolution gravity survey to elucidate the granite surface a depth which remains a target for mineral exploration and geothermal energy potential.
Despite the model by Willis-Richards and Jackson (1989) targeting the upper surface of the granite, with little constraint on deeper structure, a volume for the batholith was calculated and estimated to be of the order of 68,000 km^3^ with a thickness of 8 to 22 km.

More recent gravity modelling by Taylor (2007) confirm the tabular nature of the granite batholith but infer that the tabular emplacement model is likely to be more complicated. Furthermore, the thickness of granite plutons is revised significantly to approximately 5 to 8 km with the exception of Dartmoor at 8 to 10 km. Moreover, Shail et al. (2014) compared seismic reflection data from SWAT5 and SWAT6 (Scheirer and Hobbs, 1990) and seismic refraction data by Brooks et al. (1984) with gravity modelling of Edwards (1984) to underline that geophysical data most likely suggests a thickness to the batholith of <10 km which would result in a drastically reduced overall volume. Again, acquisition of new, high-resolution gravity measurements would enhance these interpretations.
References
Alexander, A.C., Shail, R.K., 1996. Late- to post-Variscan structures on the coast between Penzance and Pentewan, South Cornwall. Proceedings of the Ussher Society 9, 72–78.
Beer, K.E., Burley, A.J., Tombs, J.M., 1975. The concealed granite roof in south-west Cornwall. Mineral Reconnaissance Programme Report, Institute of Geological Sciences, No.1 [Unpublished].
Booth, B., Exley, C.S., 1987. Petrological features of the Land’s End Granites. Proceedings of the Ussher Society 6, 439–446.
Bott, M.H.P., Day, A.A., Masson-Smith, D., 1958. The geological interpretation of gravity and magnetic surveys in Devon and Cornwall. Philosophical Transactions of the Royal Society of London. Series A, Mathematical and Physical Sciences 251, 161–191.
Brooks, M., Doody, J.J., Al-Rawi, F.R.J., 1984. Major crustal reflectors beneath SW England. Journal of the Geological Society, London 141, 97–103. https://doi.org/10.1144/gsjgs.141.1.0097
Burley, A.J., Cornwell, J.D., Tombs, J.M.C., 1978. Geophysical field techniques for mineral exploration. Mineral Reconnaissance Programme Report. Institute of Geological Sciences. No. 20; Mineral Reconnaissance Programme Report. Institute of Geological Sciences. No. 20.
Chen, Y., Clark, A.H., Farrar, E., Wasteneys, H.A.H.P., Hodgson, M.J., Bromley, A.V., 1993. Diachronous and independent histories of plutonism and mineralization in the Cornubian Batholith, southwest England. Journal of the Geological Society, London 150, 1183–1191.
Chesley, J.T., Halliday, A.N., Snee, L.W., Mezger, K., Shepherd, T.J., Scrivener, R.C., 1993. Thermochronology of the Cornubian batholith in southwest England: Implications for pluton emplacement and protracted hydrothermal mineralization. Geochimica et Cosmochimica Acta 57, 1817–1835.
Dupuis, N.E., Braid, J.A., Murphy, J.B., Shail, R.K., Archibald, D.A., Nance, R.D., 2015. 40 Ar/ 39 Ar phlogopite geochronology of lamprophyre dykes in Cornwall, UK: new age constraints on Early Permian post-collisional magmatism in the Rhenohercynian Zone, SW England. Journal of the Geological Society 172, 566–575. https://doi.org/10.1144/jgs2014-151
Edmonds, E.A., Wright, J.E., Beer, K.E., Hawkes, J.R., Williams, M., Freshney, E.C., Fenning, P.J., 1968. Geology of the country around Okehampton. Memoir of the British Geological Survey, Sheet 324. England; Wales.
Edwards, J.W.F., 1984. Interpretations of seismic and gravity surveys over the eastern part of the Cornubian platform. Geological Society, London, Special Publications 14, 119–124. https://doi.org/10.1144/GSL.SP.1984.014.01.11
Edwards, R.A., 1999. The Minehead District - A Concise Account of the Geology. Memoir of the British Geological Survey, Sheet 278; part of 294. England; Wales.
Exley, C.S., 1996. Petrological features of the Bodmin Moor Granite, Cornwall. Proceedings of the Ussher Society 9, 85–90.
Knox, D.A., Jackson, N.J., 1990. Composite granite intrusions of SW Dartmoor, Devon. Proceedings of the Ussher Society 7, 246–250.
Leat, P.T., Thompson, R.N., Morrison, M.A., Hendry, G.L., Trayhorn, S.C., 1987. Geodynamic significance of post-Variscan intrusive and extrusive potassic magamatism in SW England. Transactions of the Royal Society of Edinburgh: Earth Science 77, 349–360.
Leveridge, B.E., Holder, M.T., Goode, A.J.J., 1990. Geology of the country around Falmouth. Memoir of the British Geological Survey, Sheet 352. England; Wales.
Leveridge, B.E., Holder, M.T., Goode, A.J.J., Scrivener, R.C., Jones, N.S., Merriman, R.J., 2002. Geology of the Plymouth and south-east Cornwall area. Memoir of the British Geological Survey, Sheet 348. England; Wales.
Manning, D.A.C., Hill, P.I., Howe, J.H., 1996. Primary lithological variation in the kaolinized St Austell Granite, Cornwall, England. Journal of the Geological Society, London 153, 827–838.
McKeown, M.C., Edmonds, E.A., Williams, M., Freshney, E.C., Masson Smith, D.J., 1973. Geology of the country around Boscastle and Holsworthy. Memoir of the British Geological Survey, Sheet 322; 323. England; Wales.
Miller, J.A., Mohr, P.A., 1964. Potassium-argon measurements on the granite and some associated rocks from SW England. Geological Journal 4, 105–126.
Miller, J.A., Shibata, K., Munro, M., 1962. The Potassium-Argon Age of the Lava of Killerton Park, near Exeter. Geophysical Journal 6, 394–396.
Powell, T., 2004. The anatomy of a pluton - a petrological investigation into the Land’s End Granite (Unpublished MPhil Thesis). University of Derby.
Prelević, D., Foley, S.F., Cvetković, V., Romer, R.L., Prelević, D., Foley, S.F., Cvetković, V., Romer, R.L., 2004. Origin of minette by mixing of lamproite and dacite magmas in Veliki Majdan, Serbia. Journal of Petrology 45, 759–792. https://doi.org/10.1093/petrology/egg109
Reid, C., Scrivenor, J.B., 1906. The geology of the country near Newquay. Memoirs of the Geological Survey, Sheet 346. England; Wales.
Roberts, C.L., 1997. The petrography of the Fremington Dyke. Proceedings of the Ussher Society 9, 182–187.
Rollin, K., 1988. A detailed gravity survey between Dartmoor and Bodmin Moor: the shape of the Cornubian granite ridge and a new Tertiary basin. Proceedings of the Geologists’ Association 99, 15–25. https://doi.org/10.1016/S0016-7878(88)80024-5
Rollin, K.E., O’Brien, C.F., Tombs, J.M.C., 1982. Seismic and gravity surveys over the concealed granite ridge at Bosworgy, Cornwall. Mineral Reconnaissance Programme Report, Institute of Geological Sciences, No. 49; Mineral Reconnaissance Programme Report, Institute of Geological Sciences, No. 49.
Rundle, C.C., 1981. K-Ar ages for micas from SW England. Institute of Geological Sciences, Isotope Geology Unit Report, No. 81⁄10.
Rundle, C.C., 1980. K-Ar ages for lamprophyre dykes from SW England. Institute of Geological Sciences, Isotope Geology Unit Report, No. 80⁄9.
Scheirer, D.S., Hobbs, R.W., 1990. Seismic attenuation in the continental crust SW of England. Geophysical Journal International 103, 533–540. https://doi.org/10.1111/j.1365-246X.1990.tb01789.x
Scrivener, R.C., 2006. Cornubian granites and mineralization of SW England, in: Brenchley, P.J., Rawson, P.F. (Eds.), The Geology of England and Wales. The Geological Society, London, pp. 257–268.
Shail, R.K., Leveridge, B.E., 2009. The Rhenohercynian passive margin of SW England: Development, inversion and extensional reactivation. Comptes Rendus Geoscience 341, 140–155.
Shail, R., Scrivener, R., Simons, B., Müller, A., Andersen, J., Williamson, B.J., Halls, C., Hughes, S., 2014. Early Permian post-Variscan magmatism, in: Shail, R., Andersen, J., Simons, B., Williamson, B.J. (Eds.), EUROGRANITES 2014: Granites and Mineralisation of Sw England. Field Excursion Guidebook 12th-18th July 2014, pp. 14–36.
Simons, B., Andersen, J.C., Shail, R.K., Jenner, F., 2017. Fractionation of Li, Be, Ga, Nb, Ta, In, Sn, Sb, W and Bi in the peraluminous Early Permian Variscan granites of the Cornubian Batholith: precursor processes to magmatic-hydrothermal mineralisation. Lithos 278-281, 491–512. https://doi.org/10.1016/j.lithos.2017.02.007
Simons, B., Shail, R.K., Andersen, J.C., 2016. The petrogenesis of the Early Permian Variscan granites of the Cornubian Batholith: Lower plate post-collisional peraluminous magmatism in the Rhenohercynian Zone of SW England. Lithos 260, 76–94. https://doi.org/10.1016/j.lithos.2016.05.010
Tapster, S., 2019. Geochronology in SW England. [in preparation]
Taylor, G.K., 2007. Pluton shapes in the Cornubian Batholith: new perspectives from gravity modelling. Journal of the Geological Society, London 164, 525–528.
Tombs, J.M.C., 1980. Results of a gravity survey of the south-west margin of Dartmoor, Devon. Mineral Reconnaissance Programme Report, Institute of Geological Sciences, No. 34.
Tombs, J.M.C., 1977. A study of the space form of the Cornubian granite batholith and its application to detailed gravity surveys in Cornwall. Mineral Reconnaissance Programme Report, Institute of Geological Sciences, No. 11; Mineral Reconnaissance Programme Report, Institute of Geological Sciences, No. 11.
Willis-Richards, J., Jackson, N.J., 1989. Evolution of the Cornubian Ore Field, Southwest England: Part I. Batholith Modeling and Ore Distribution. Economic Geology 84, 1078–1100.